The Wonders of Electromagnetism
Understanding Magnetization and Demagnetization
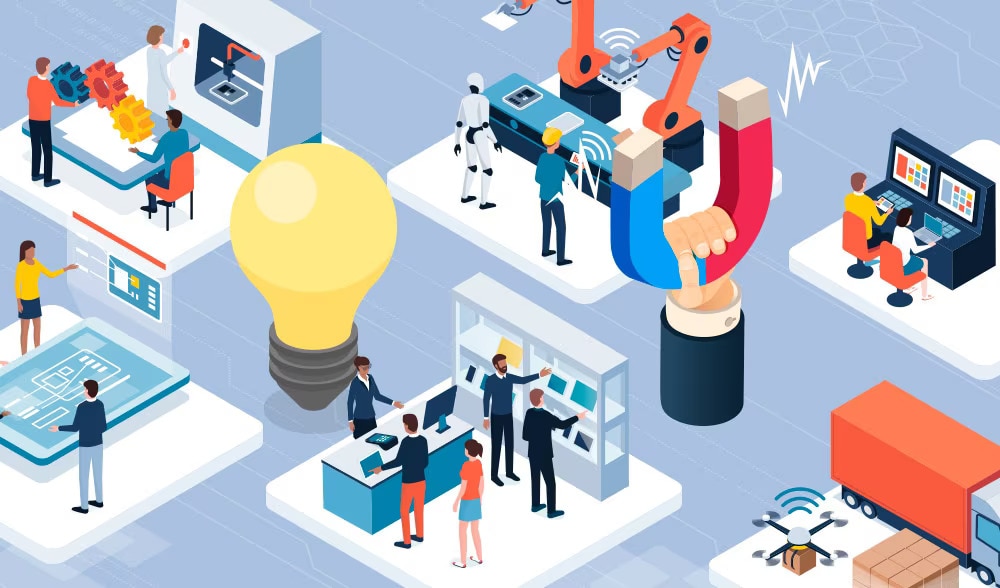
Magnetite becomes a natural magnet through magnetization
A lodestone is a natural magnet powerful enough to attract materials like ironsand and nails. Its name is said to derive from the old English meaning of lode as “course” or “lead,” referring to the utility of a natural magnet as a compass that guides travelers to their destinations.
For a natural magnet to form, a strong magnetic field must be present in the external environment. The first possibility that may come to mind is geomagnetism, but Earth’s geomagnetic field is too weak to magnetize a material strongly enough so it can attract ironsand or nails. One popular explanation is lightning. When lightning strikes, a large electrical current flows across the ground, which creates a strong magnetic field around its path. This process is believed to be how magnetite (an iron ore mineral) found inside rocks becomes strongly magnetized.
Note that “magnetite” here refers to magnetite in a broad sense. In strict mineralogical terms, magnetite is a substance that sticks to magnets but cannot be magnetized enough to become a natural magnet itself. Only when magnetite transforms into a mineral known as maghemite through weathering and oxidization can it be strongly magnetized and remain so. In other words, magnetite is not to be equated with natural magnets; rather, natural magnets should be regarded as a special type of transformed magnetite.
Ironsand also sticks to magnets but does not remain strongly magnetized. While ironsand is commonly understood to be magnetite particles, it is actually an iron titanium oxide. A substance in which two or more solids are uniformly mixed, like a metal alloy, is known as a solid solution. Ironsand is a collective term describing a continuous solid solution of iron oxides and titanium oxides in varying proportions (for example, traditional Japanese swords are made of high-quality ironsand with low titanium content). Iron oxides comprise a vast physical world with a broad spectrum of compositions and crystal structures. This is why ferrite, primarily composed of iron oxides, has a nearly infinite variety of compositions and properties.
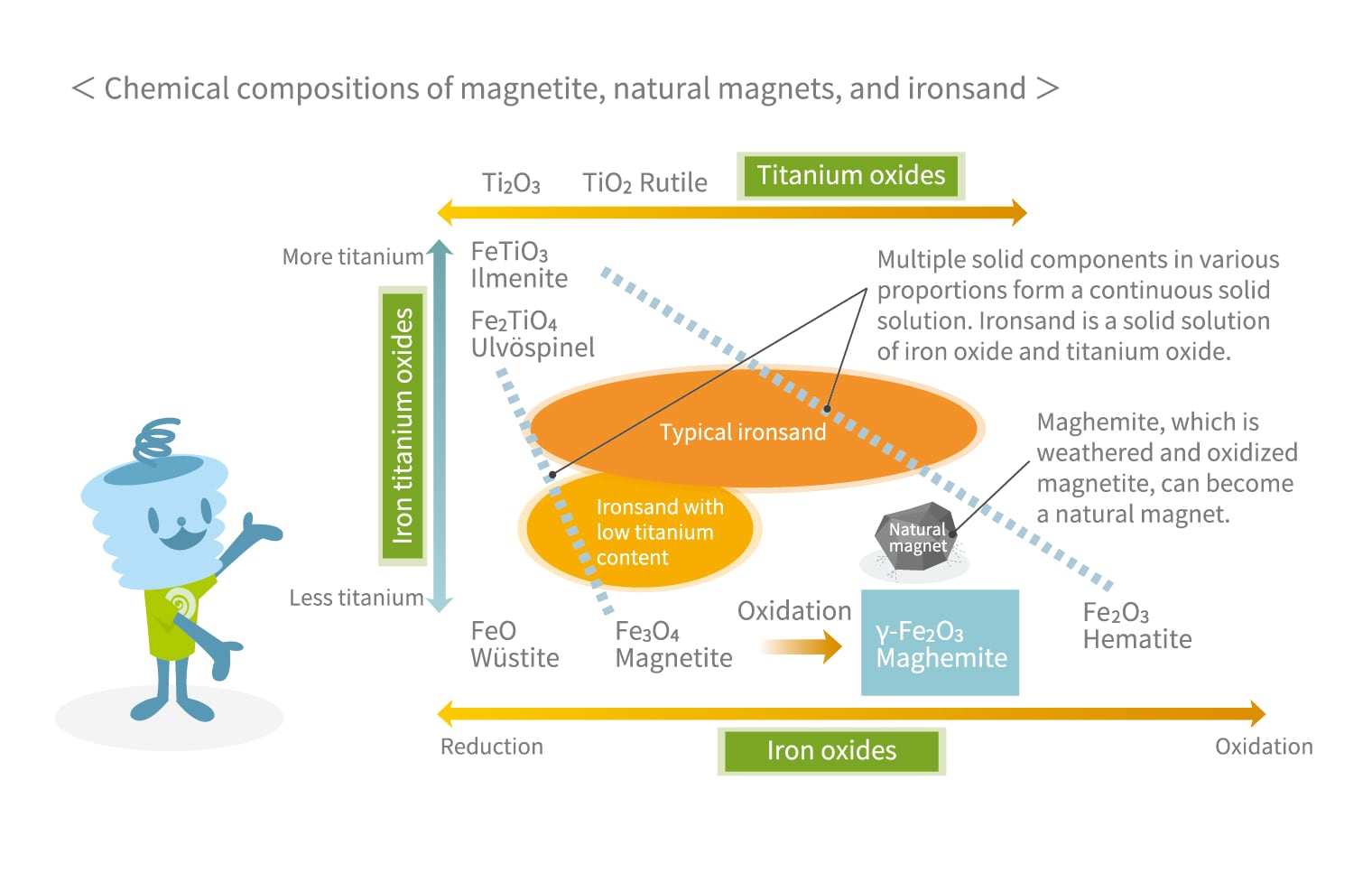
What does “magnetization” mean?
When we think of a magnet, we think of attraction to iron. However, magnets are not born with this capability. Only when a magnetic material (a material that possesses the properties necessary to become a magnet) is exposed to magnetic forces does it turn into a magnet and gain the ability to attract iron. This process is called magnetization.
Multipole magnetization enables compact, thin-profile, high-performance motors
It follows from the discussion thus far that industrially produced magnets are not magnetized from the beginning. They are first formed from a magnetic material, then placed in a device called a magnetizer that applies a powerful magnetic field. It is only then that the material comes to life as a magnet.
The simplest magnetizer is based on a solenoid (cylindrical) coil. When a magnetic material is placed in the coil, and a direct current is passed through the coil, the magnetic field projecting out of the coil magnetizes the material. Since the process requires current to flow for only a brief moment, expending a continuous current for this purpose would be a waste of power. Instead, a large capacitor is used to store an electric charge, which is instantaneously discharged into the coil, momentarily creating a powerful magnetic field. This is similar to how a camera’s strobe works: a charge stored in the built-in aluminum electrolytic capacitor is discharged into the lamp all at once, setting off an intense flash of light. Because magnetizers require a much greater current (several kA to 10 kA or more), huge capacitors (oil capacitors and chemical capacitors) rated at several hundred to several tens of thousands of μF (microfarads) are used.
Magnets inside small brushless motors, commonly found in home appliances, are magnetized in complex patterns. For example, DVD recorders and hard disk drives (HDDs) in personal computers read and write information on disks spinning at high speeds. Thin-profile motors, known as spindle motors, are used for this high-speed rotation. There are various types of spindle motors, one of which is a brushless motor with an outer rotor. In this type of motor, an electromagnet with gear-shaped, salient poles serves as the stator, and a surrounding ring magnet spins with the rotor. The ring magnet also features multiple poles. Pairing it with a salient-pole electromagnet ensures smooth, stable motor rotation. Multipole magnets are magnetized using a special iron core called a yoke that matches the desired magnetization pattern.
Ring magnets designed for DVD and HDD spindle motors are bonded magnets, produced by molding a mixture of powdered magnets (e.g., powerful neodymium magnets) and plastic. Although mixing with plastic reduces the magnetic force, it provides the freedom and precision to form complex, thin-walled shapes. Multipole magnetization using custom yokes has enabled the widespread utilization of compact, thin-profile, high-performance motors in familiar devices around us.
TDK has consistently embraced challenges over the past half-century, starting with the development of the FB1A ferrite magnet material in 1959 to state-of-the-art neodymium magnets and on to the world’s first La-Co high-performance ferrite magnet, contributing substantially to the advancement of modern industry.
TDK offers a lineup of high-performance neodymium magnets that are completely free of heavy rare earth elements such as Dy (dysprosium) and Tb (terbium), as well as those produced using the heavy rare earth diffusion (HAL) method, which results in a significant reduction of the amount of heavy rare earth elements used compared to conventional processes.
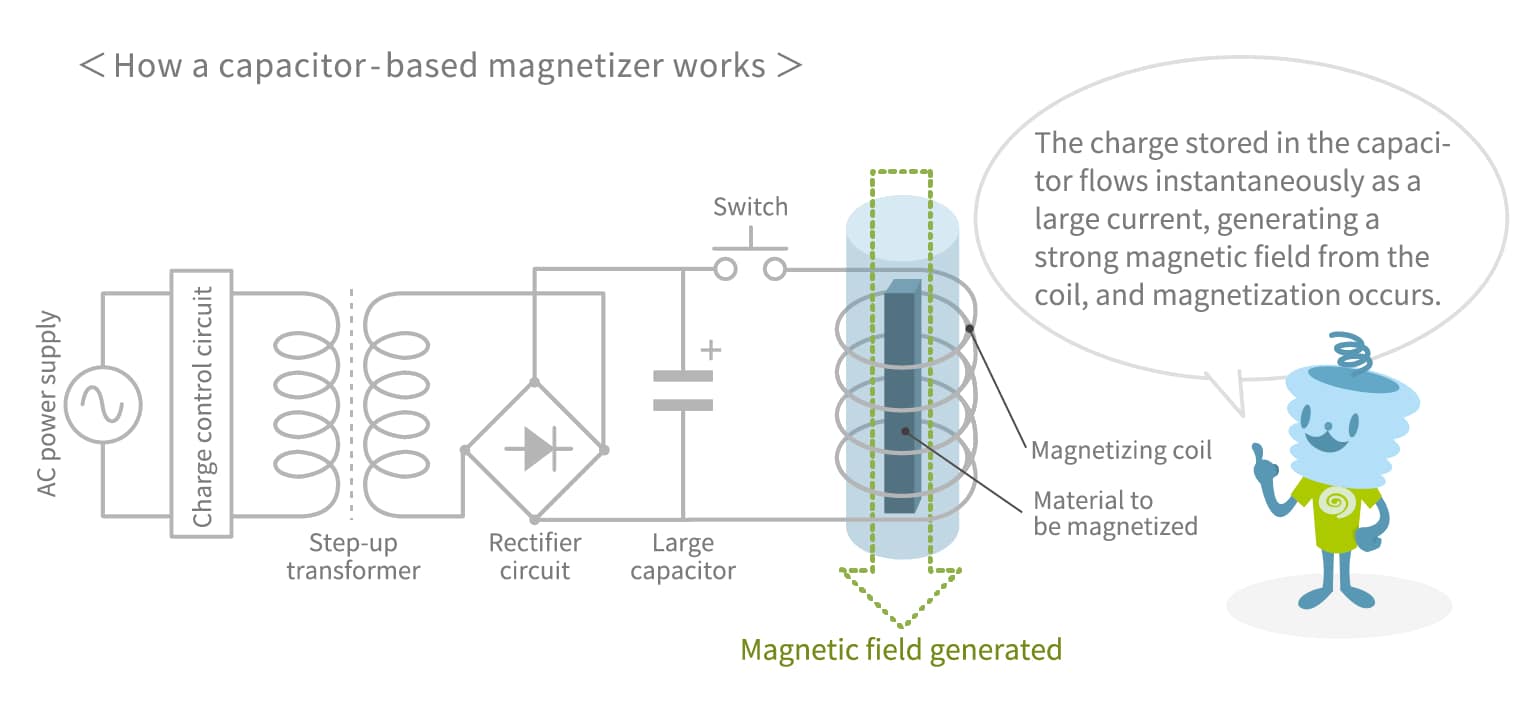
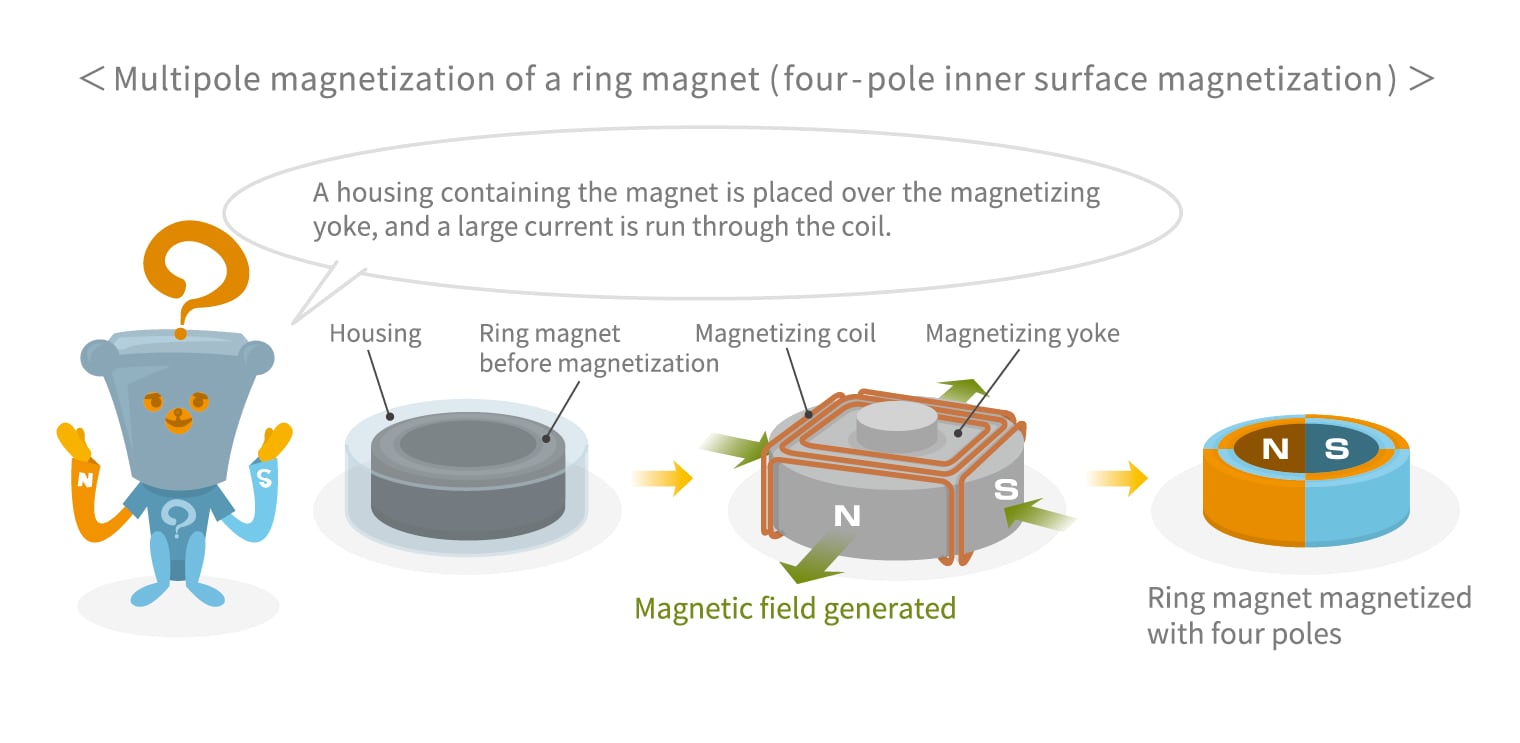
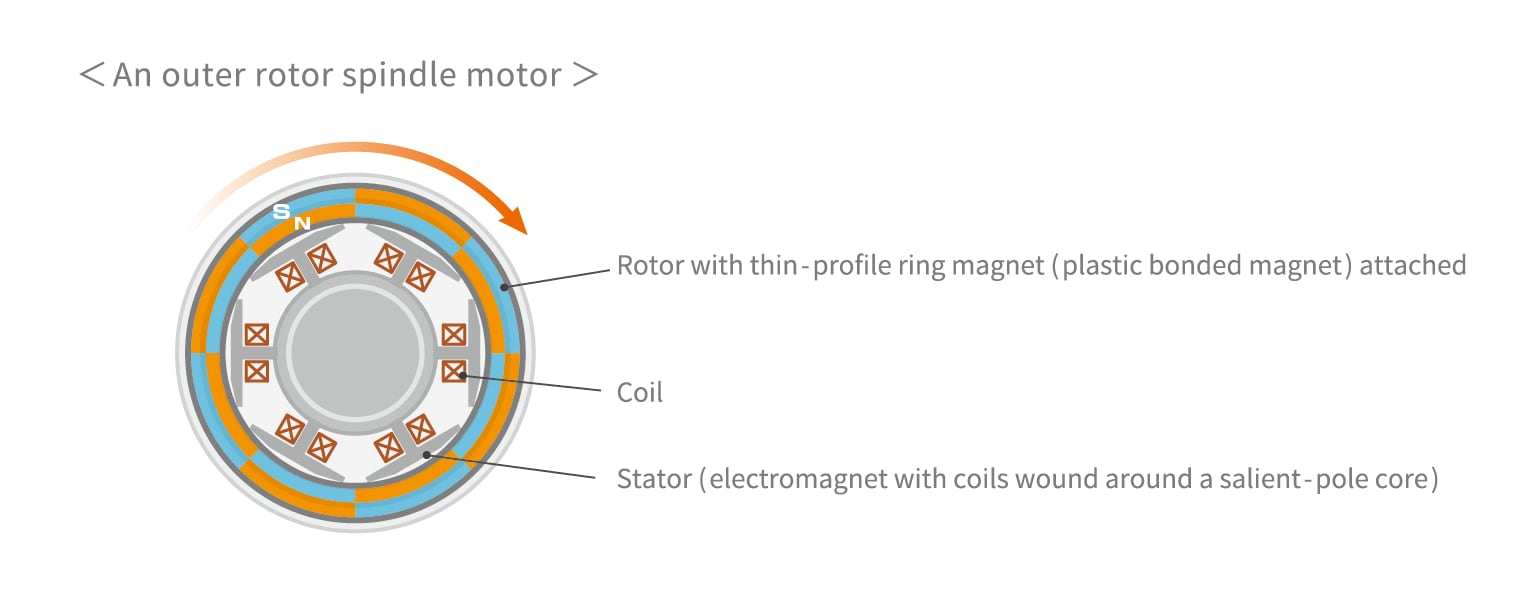
AC demagnetization and how it works in VCR erase heads
The process of returning a magnet to its original, unmagnetized state is called degaussing or demagnetization. The simplest method is thermal demagnetization. When an external magnetic field is applied to a magnetic material, it transforms into a magnet as the multitude of “mini-magnets” inside align their magnetic poles uniformly. However, when heated above a certain temperature (known as the Curie temperature), the orientation of these “mini-magnets” becomes chaotic, causing the material to lose its magnetism as a whole. This explains why red-hot iron does not stick to magnets.
Tape recorders and VCRs erase recorded signals on magnetic tape using a method known as AC (alternating current) demagnetization. This is achieved by running an alternating current—large enough to cause magnetic saturation in the magnetic powder on the tape—through the erase head’s coil. The alternating magnetic field projecting from the head reverses the polarity of the magnetic powder repeatedly. However, as the tape travels and moves away from the head, the effect of the head’s magnetic field diminishes, and so does the degree of magnetization of the magnetic powder. The combined effect of the repetitive reversal of polarity and the gradual decay of magnetization returns the magnetic powder to its original, unmagnetized state.
AC demagnetization can also be demonstrated using commercial AC power. Wrap a wire around a plastic tube to create a coil, connect it to a variable transformer, and place a magnet inside the coil. Set the variable transformer’s dial to around 20 to 30 volts, plug it into an outlet, and slowly turn the dial down to zero. The coil will generate an alternating magnetic field that continues reversing in polarity at the AC power’s frequency (usually 50 or 60 Hz), which gradually weakens as the transformer is dialed down, finally demagnetizing the magnet. The underlying principle is identical to that of the tape recorder’s erase head.
Instead of using AC, some types of demagnetizers utilize the resonance phenomenon between a capacitor and a coil. When the charge stored in a capacitor is discharged into a coil, the coil generates a current in the opposite direction, which recharges the capacitor. The capacitor discharges again, and the cycle repeats itself. Due to the electrical resistance of the coil and other components, the resonance dissipates naturally, creating the same effect as AC demagnetization and returning a magnet to its unmagnetized state.
When a screwdriver is left stuck on a magnet, the screwdriver becomes magnetized. This can be useful for picking up small screws with the screwdriver but can be inconvenient if the screws also become magnetized. Removing the unwanted magnetization of tools and components is one use of a demagnetizer.
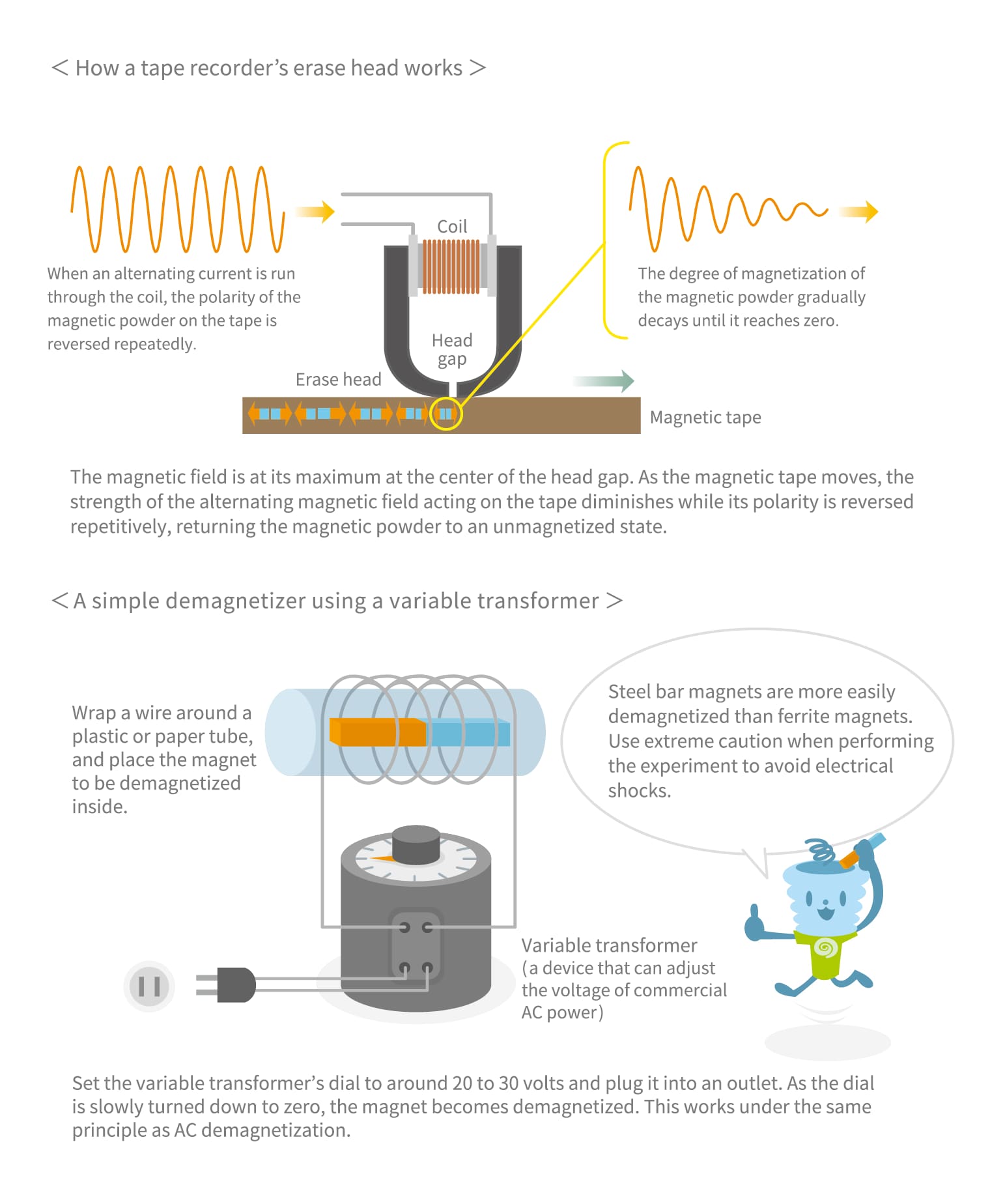
Magnetization and demagnetization have promising prospects
Even today, technologies based on magnetism are being deployed in an ever-increasing number of scenarios. Efficiency improvements in renewable energy sources like wind power, higher-performance EV motors, and advances in magnetic sensors and magnetic resonance imaging (MRI) are just a few examples of innovations powered by magnetism that are enriching our lives. Demagnetization techniques, which remove unwanted magnetism, also play essential roles in diverse fields, including information security, manufacturing, and defense. Human ingenuity in controlling magnetism will surely attract the development of next-generation electronics.
TDK is a comprehensive electronic components manufacturer leading the world in magnetic technology